AIM Science Highlights
–2 November 2012–
1. Momentum budget of the migrating diurnal tide
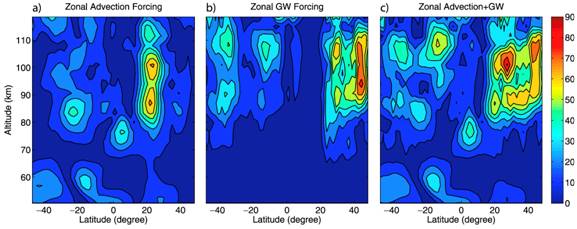
An analysis of the momentum budget of the diurnal westward-propagating wavenumber-1 (DW1) tide simulated by the Whole Atmosphere Community Climate Model (WACCM) found that momentum advection makes a dominant contribution to the phase change of DW1 in the zonal wind compared with the effects of gravity-wave forcing. The figure shows the Time tendency amplitudes in the DW1 component of (a) total advection forcing, (b) total gravity-wave forcing and (c) the sum of them in the zonal momentum from WACCM simulation, in units of m/s/day.
Reference: Lu, X., H.-L. Liu, A. Z. Liu, J. Yue, J. M. McInerney, and Z. H. Li, Momentum budget of the migrating diurnal tide in the Whole Atmosphere Community Climate Model, J. Geophys. Res., 117, D07112, doi:10.1029/2011JD017089, 2012.
2. Solar-flare effects on ionospheric electrodynamics.
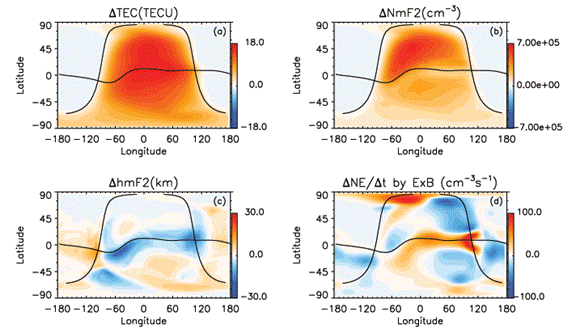
The sudden increase of X-ray and extreme ultra-violet irradiance during flares increases the density of the ionosphere through enhanced photoionization. Model simulations showed that additional ionospheric changes come from electrodynamics, with a change in the vertical E×B drift in the magnetic equatorial region playing a significant role. The figure shows horizontal maps of the ionosphere responses to the X17 flare that occurred on October 28, 2003 at 11:10 UT, about 20 minutes after the peak of the flare. (a) change of total electron content (TEC); (b) change of peak ionospheric density NmF2; (c) change of the height of the peak density hmF2; and (d) rate of change of electron density due to vertical E×B drifts at pressure level +2 (approximately 250 km altitude). Also plotted in the figure are the terminator and magnetic equator.
Reference: Qian, L., A. B. Burns, S.C. Solomon, and P.C. Chamberlin (2012a), Solar flare impacts on ionospheric electrodynamics, Geophys. Res. Lett., 39, L06101, doi:1019/2012GL051102.
3. Ionospheric storm effects of disturbed electric fields.
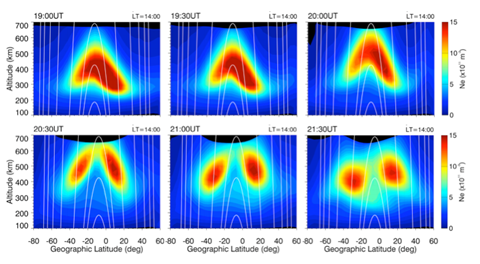
A comprehensive modeling investigation of ionospheric and thermospheric variations during a prompt penetration electric field on 9 November 2004 showed that the TIME-GCM is able to successfully reproduce the large vertical ion drift of ~120 m/s over the Jicamarca incoherent radar in Peru, and to reveal dramatic changes in ionospheric density that occur during a storm. The figure shows altitude distributions of electron density at selected UT times on 9 November 2004 that reveal the super-fountain effect associated with the prompt penetration electric fields. The white lines indicate geomagnetic field lines.
Reference: Lu, G., L. Goncharenko, M. J. Nicolls, A. Maute, A. Coster, and L. J. Paxton (2012), Ionospheric and thermospheric variations associated with prompt penetration electric fields, J. Geophys. Res., 117, A08312, doi:10.1029/2012JA017769.
4. Global ionospheric climatology.
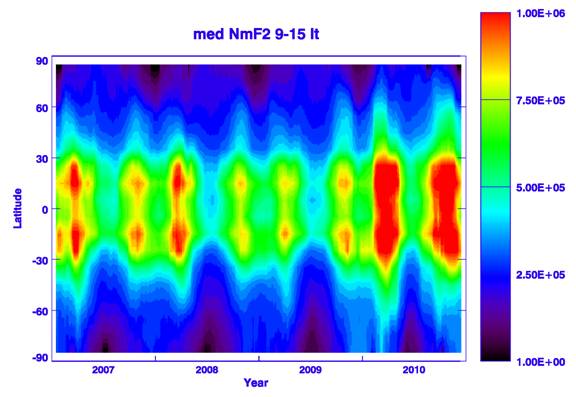
Extensive analyses of COSMIC data for 2007-2010 have revealed a number of features concerning seasonal and solar-cycle variation. Low-latitude ionospheric peak density NmF2 was dominated by the semi-annual anomaly, the equatorial anomaly and the annual asymmetry. The second equinoctial maximum is not centered on the September equinox, but occurred in October. There is an annual variation at high latitudes in which maximum values of NmF2 occur in summer, unlike analyses of earlier periods that showed a winter anomaly. Elevated height of the peak, hmF2, also occurs in summer at high latitudes, with a distinct seasonal and hemispheric asymmetry. The figure shows NmF2 climatology for 2007 to 2010 in magnetic latitude bins. Units are in per cubic centimeters. Each bin is calculated using the median of data from all longitudes for local times between 0900 and 1500 local solar time. The data were then further processed by taking a 30 day running median for each bin to remove short term variability like geomagnetic activity and any possible short term “breathing” modes of the atmosphere.
Reference: Burns, A.G., S.C. Solomon, W. Wang, L. Qian, Y. Zhang, and L.J. Paxton (2012a), Daytime climatology of ionospheric NmF2 and hmF2 from COSMIC data, J. Geophys. Res., 117, A09315, doi:10.1029/2012JA017529.
5. Impact of high-speed streams and co-rotating interaction regions on the thermosphere-ionosphere.
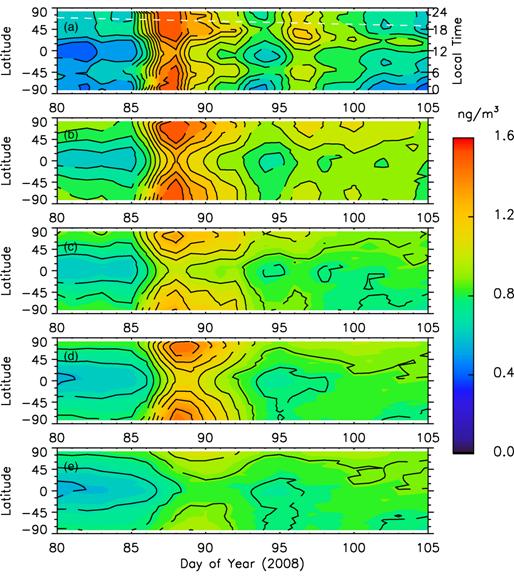
Changes in the thermosphere-ionosphere system caused by high-speed streams in the solar wind, and the co-rotating interaction regions they engender, were studied using a combination of CMIT and TIEGCM simulations and data analysis. It was found that the interplanetary magnetic field (IMF) is more important than solar wind speed and density per se in controlling magnetosphere-ionosphere coupling. Numerical experiments were conducted to elucidate the mechanisms of solar wind and IMF forcing. The figure shows a comparison of thermospheric neutral density at 400 km measured by the STAR accelerometer on the CHAMP satellite with TIE-GCM simulations for the Whole Heliosphere Interval (WHI) during March-April 2008. (a) CHAMP observations from the ascending node, near 21–18 local solar time as indicated by the white dashed lines. (b) Simulations at the local time and latitude of the satellite using the TIE-GCM, with high-latitude forcing derived from the Weimer 2005 model using all measured solar wind and IMF parameters. (c) Simulations with the solar wind speed set to 400 km s-1 and density set to 4 cm-3. (d) Simulations with the IMF y and z components smoothed using a running 72-hour centered mean. (e) Simulations with the IMF y and z components set to zero.
Reference: Solomon, S. C., A. G. Burns, B. A. Emery, M. G. Mlynczak, L. Qian, W. Wang, D. R. Weimer, and M. Wiltberger (2012), Modeling studies of the impact of high-speed streams and co-rotating interaction regions on the thermosphere-ionosphere, J. Geophys. Res., 117, A00L11, doi:10.1029/2011JA017417.
6. Dependence of solar-wind/magnetosphere/ionosphere coupling on the geomagnetic dipole strength.
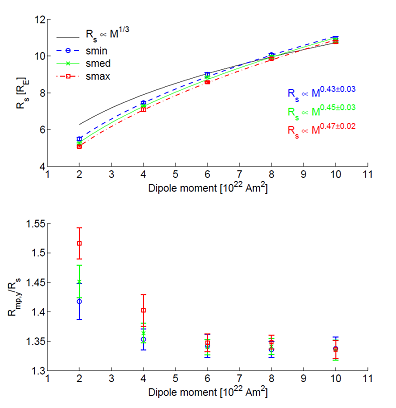
The possible effects of geomagnetic-field changes over periods of millennia on solar wind/magnetosphere/ionosphere/thermosphere coupling was examined, by altering the strength of the geomagnetic dipole in the Coupled Magnetosphere-Ionosphere-Thermosphere (CMIT) model. These changes affect ionospheric conductivity and magnetosphere-ionosphere coupling. The figure shows the stand-off distance Rs (top) and the ratio between the stand-off to the flank and nose of the magnetosphere (bottom) as a function of dipole moment for solar minimum (blue), medium (green) and maximum (red) conditions. The black line indicates the theoretical relationship Rs µ M1/3, where M is the geomagnetic dipole moment.
Reference: Cnossen, I., A. D. Richmond, and M. Wiltberger (2012a), The dependence of the coupled magnetosphere-ionosphere-thermosphere system on the Earth's magnetic dipole moment, J. Geophys. Res., 117, A05302, doi:10.1029/2012JA017555.
7. Driving magnetosphere-ionosphere coupling in upper-atmosphere models with observed field-aligned currents from the AMPERE mission.
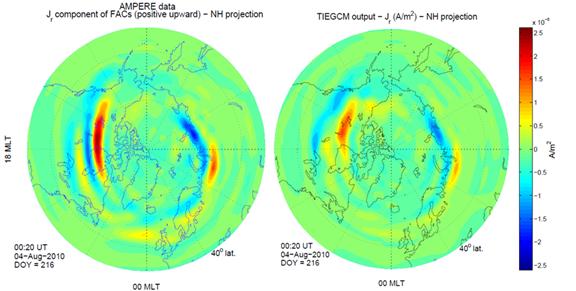
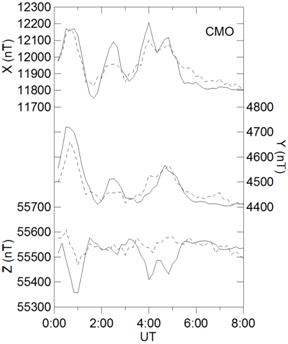
A significant development for the the NCAR Thermosphere-Ionosphere-Electrodynamics General-Circulation Model (TIEGCM) was the addition of the capability to specify the electrodynamical coupling of the ionosphere with the magnetosphere by using observations of geomagnetic-field-aligned currents from the multi-satellite AMPERE project. This was shown to drive ionospheric currents in the TIEGCM that produce magnetic perturbations at the ground comparable to observations in the auroral regions for specific events. The figure shows: (Left) Observed field-aligned currents in the northern hemisphere from AMPERE, compared with the currents calculated from the TIEGCM when the sum of northern and southern AMPERE currents are used to drive the electrodynamics; (Right) Comparison between simulated (dashed) and observed (solid) ground magnetic perturbations at College, Alaska.
Reference: Marsal, S., A.D. Richmond, A. Maute, and B.J. Anderson (2012), Forcing the TIEGCM model with Birkeland currents from the Active Magnetosphere and Planetary Electrodynamics Response Experiment, J. Geophys. Res., 117, A06308, doi:10.1029/2011JA017416.
8. Lunar tide effects on the ionosphere during a stratospheric sudden warming.
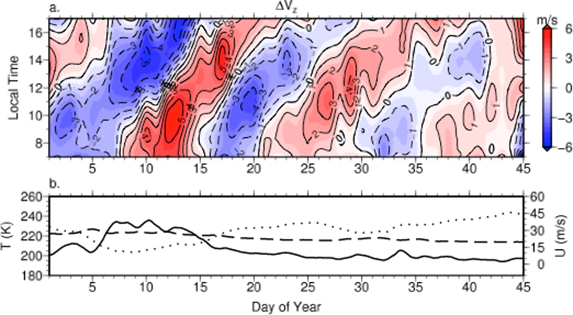
Lunar tides occur in the atmosphere due to the combination of direct lunar tidal forcing and to vertical movement of the Earth/ocean surface at lunar tidal periods. The effects are noticeable in the upper atmosphere, and appear to be significantly amplified during stratospheric sudden warmings. Lunar tidal forcing was added to the Whole-Atmosphere Community Climate Model (WACCM), and the resultant lunar variations in ionospheric vertical drifts were shown to be realistically modeled. The figure shows: (a) Change in the zonal mean vertical drift velocity at the magnetic equator and 300 km. The zonal means are calculated at fixed local times. The changes are with respect to the average local time variation in the vertical drift velocity over the interval shown. (b) 10 hPa daily zonal mean temperature at 90°N (solid) and 60°N (dashed), and zonal wind at 60°N (dotted). Results are for solar minimum.
Reference: Pedatella, N.M., H.-L. Liu, A.D. Richmond, A. Maute, and T.-W. Fang (2012c), Simulations of solar and lunar tidal variability in the mesosphere and lower thermosphere during sudden stratosphere warmings and their influence on the low-latitude ionosphere, J. Geophys. Res., 117, A08326, doi:10.1029/2012JA017858.
–31 July 2012–
1. Balloon-borne Sensor Finds Equatorward Thermospheric Wind
Using High-Altitude Interferometer Wind Observation (HIWIND), a balloon-borne interferometer, Wu et al. (2012) measured the observed wavelength of airglow given off by excited atomic oxygen, which peaks in intensity in the upper thermosphere at 250-km altitude. The light emitted from atomic oxygen has a consistent wavelength of 630 nm. So by looking for deviations from this value due to the Doppler effect, the authors were able to calculate the relative motion of the source oxygen atoms and thus the speed and direction of the thermospheric wind.
Figure Caption: HiWind Neutral Wind and EISCAT Ion Drift2011165.
Geophysical Research Letters »
–14 December 2011–
1. Modeling the Impact of Severe Space Weather
Space physicist, Michael Wiltberger, from the High Altitude Observatory at the National Center for Atmospheric Research, said that new model simulations show for the first time that out-flowing oxygen ions may play an important role in how the magnetosphere responds to the fluxes of energetic, charged particles from severe space weather.
Figure Caption: The Coupled Magnetosphere Ionosphere Thermosphere model will offer an improved view on the dynamic exchanges of charged materials generated during magnetic storms and the resulting effects on Earth's technological systems.