[History]
[Methods]
[Planets Found]
[Return to Main Page]
This page is obsolete and contains many broken links
History of the Search
Early History
Pondering on the existence of worlds and solar systems other than our own is
not new. It goes back as far as ancient times, perhaps even further. The
Greek atomists
(1,
2)
in the fifth century B.C. comtemplated the formation of worlds other than our
own:
The worlds come into being as follows: many bodies of all sorts and shapes
move from the infinite into a great void; they come together there and produce
a single whirl, in which, colliding with one another and revolving in all manner
of ways, they begin to separate like to like.
Leucippus
(~480-420 B.C.)
While many ancient scientists and philosophers were interested in the formation
of planets within our own solar system, believing perhaps that ours was unique
in the universe, others considered the possibility of countless worlds of
infinite variety:
In some worlds there is no Sun and Moon, in others they are larger than in
our world, and in others more numerous. In some parts there are more worlds,
in others fewer (...); in some parts they are arising, in others failing.
There are some worlds devoid of living creatures or plants or any moisture.
Democritus (~460-370 B.C.)
There are infinite worlds both like and unlike this world of ours. For the
atoms being infinite in number, as was already proven, (...) there nowhere
exists an obstacle to the infinite number of worlds.
Epicurus
(341-270 B.C.)
Unfortunately, the views of the atomists were ahead of their time. The work
of the great philosopher Aristotle did much to overshadow the advancement of
thought on this subject. He staunchly believed in a geocentric universe.
The Earth was the center, the only world to contain life in an otherwise
unchanging celestial sphere:
There cannot be more worlds than one.
Aristotle (384-322 B.C.)
Aristotle's views prevailed through much of the next two thousand years.
During the Renaissance, the existence of other worlds became mainly a religious
quandary. Since there was no way to prove it either way, the scriptures became
the guidebook. It was thought that God could create other worlds. The
question was whether He would choose to.
The reopening of science's eyes came in the sixteenth century with the
introduction of the heliocentric universe theory of
Nicolaus Copernicus in 1543. One of his contemporaries, the Italian
philospher
Giordano Bruno professed his belief in an infinite number of stars,
which have an infinite number of terrestrial worlds orbiting them.
His beliefs were not shared, however. He was burned at the stake during the
Inquisition.
The invention of the telescope (or rather the retooling of a Dutch-invented
spyglass) by
Galileo in
1609 resulted in the discovery of the true nature of the Moon and planets.
He also found that the Milky Way was composed of thousands-upon-thousands of
stars too faint to resolve with the unaided eye. Although Galileo also had
some trouble with the church, his discoveries opened the minds and eyes of
subsequent generations of scientists and philosophers. Now the actual search
for extrasolar planets could begin (at least after the telescopic discovery
of the remaining planets in our own solar system:
Uranus by William Herschel in 1781,
Neptune by Galle and d'Arrest in 1846, and
Pluto by Clyde Tombaugh in 1930).
Recent History
The first documented (see
reference, PS file) search for extrasolar planets was by
Christian Huygens
(1629 - 1695) in the late seventeenth century. Although there were most likely
other early attempts at this elusive goal, the documentation is extremely
scarce.
In the mid-twentieth century came the first reported extrasolar planets.
Astronomer Peter van de Kamp pored over decades worth of photographic plates
charting the proper motion of
Barnard's Star. He found that this charting, called astrometry,
indicated a slight wobble in the star's path. After further calculation, he
arrived at the conclusion that the wobble was caused by a planet of around
1.6 times the mass of Jupiter in an eccentric orbit (see
reference).
Over the next couple of decades van de Kamp refined his calculations so that,
by 1982, he asserted that there were two planets in circular orbits around
Barnard's Star with masses of 0.7 and 0.5 that of Jupiter. His 'planets' were
never confirmed, but not for lack of trying. Many astronomers tried to verify
van de Kamp's work, only to find that there was either no wobble at all or a
wobble caused by discrepancies in the methods used to produce the photographic
plates. Peter van de Kamp died in 1995 still standing by his beliefs.
Where are we now?
Confirmed extrasolar planets and the methods of their discovery are discussed
in the next two sections, Methods and
Planets Found.
top of page
Search Methods
Pulsar Timing
At the end of their lives, stars with masses of around 15 to 30 times the
mass of our Sun explode in a supernova and leave behind a remnant called a
neutron star (masses less than this threshhold leave behind white
dwarves while greater masses lead to the formation of black holes). Sometimes
the magnetic field and spin axis of the neutron star are not aligned. As the
star spins, a 'beacon' of radio waves hit the Earth at regular intervals --
usually between 2 milliseconds to a couple of seconds. These special
neutron stars are called pulsars. Pulsars' signals are extremely regular,
with a typical rate of change of only a second per ten million years.
Because of this, small anomalies in the timing of pulsars can betray the
existence of planets orbiting the now-dead star. Planets with masses on
order of the Earth's or greater can be detected. The first extrasolar
planets confirmed were found using this method (by Wolszczan and Frail in
1992,
reference). It is currently thought that any planets found around
pulsars are likely to have formed after the supernova, as any planets in
the system before the star went nova would likely be destroyed.
Radial Velocity Measurement
A planet orbiting around its parent star exerts a pull that causes the star
to 'wobble' around the center of mass between the two objects.
If the system is oriented nearly edge-on to the Earth, the movement of the
star toward and away from the observer will cause its light to be slightly
blue and red shifted respectively (see figure). Telescopes outfitted with
precise spectrometers can measure this small shift with respect to dark
absorption lines in the spectrum. For this reason, radial velocity measurement
is also known as doppler spectroscopy.
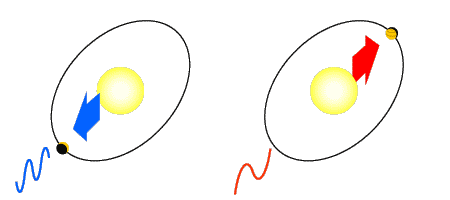
Figure based on one by G. Esquerdo of the
Arizona Search for Planets Project.
Because the motion of the star caused by orbiting planets is so small,
this method is best suited for finding large planets in tight orbits. For a
solar-mass star, a planet at a distance of 1 AU with an orbit oriented exactly
edge-on to the observer would need a mass of at least 67 times the mass of the
Earth to be detected. However, the vast majority of the exoplanets
discovered thus far were found using radial velocity measurements, including
the so-called 'hot Jupiters' -- gas giant planets in tight orbits countering
previous planet-formation theories. One of the most successful implementors
of the radial velocity technique is the team headed by Geoff Marcy, with
multiple planets discovered.
Marcy also happens to be a STARE Project
collaborator.
Astrometry
As mentioned above, as a planet revolves around its star, it pulls the star
around through its orbit. If the system is oriented face-on and the
orbiting planet is massive enough, this small motion of the star can be
detected by astrometry. As seen in the figure below, as the planet moves
through its orbit (red dots), the star revolves around the system's center of
mass, called the barycenter (the black cross). In an actual planetary system,
the star's mass is so great compared to the planet's, that the barycenter will
most likely lie within the star itself, and the path in which it moves (blue
dots) will be a much smaller circle. Astrometry, therefore, relies on very
precise measurement of a star's position.
Gravitational Lensing
Gravitational lensing occurs when a background object's light is bent due to
the gravity of the foreground object. In the past, this effect was used to study
light from dim, distant galaxies as their light was bent around closer ones.
Recently, however, sensitive equipment has been used to observe lensing events
from stars in the hub of our own galaxy. A lightcurve from a stellar lensing
is generally smooth, so when a companion planet of sufficient brightness also
moves behind the 'lens', a perturbance in the lightcurve results (see figure
below).
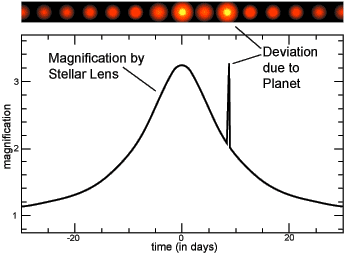
Figure from Microlensing Planet Search Project.
Photometry
If an extrasolar planetary system is oriented so that Earth lies near the
plane of the planet's orbit, then once per orbit the planet passes between its
star and the Earth, causing a transit. Using telescopes coupled with
sensitive CCD cameras, the miniscule decrease in light caused by these transits
can be measured. See the STARE
Method Page.
top of page
Extrasolar Planets Found
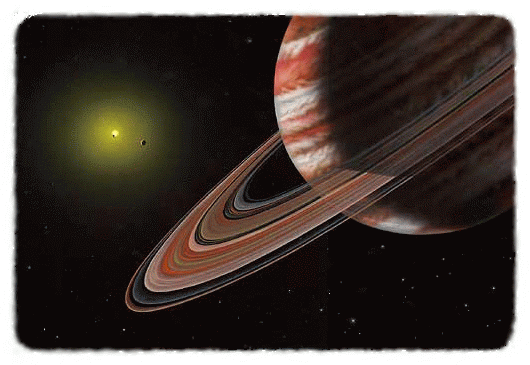
Illustration of the
Upsilon Andromedae planetary system
© 1999 Lynette Cook, all rights reserved, used with
permission of the artist.
This is an exciting time. There are so many new planets being discovered that
it is nearly a full-time job to keep track of them all! Instead of doing
a poor reporting job, we instead refer you to the Extrasolar Planets Encyclopaedia's Extrasolar Planets
Catalog: The most up-to-date listing of extra-solar planets on
the web.
top of page